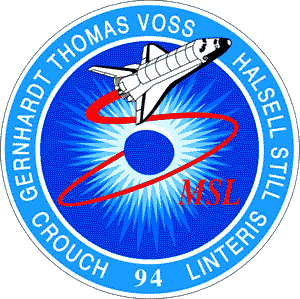
STS-94/MSL-1 Mission
DCE and FSDC-2 Initial Summaries
Mission Notes
The shuttle STS-94 mission launched on July 1, 1997 was a very special mission in that it was a reflight of the MSL-1 laboratory mission originally flown on STS-83in April 1997. The same personnel and experiments were flown, and both the Droplet Combustion Experiment (DCE) and the Fiber Supported Droplet Combustion (FSDC-2) experiments operated on STS-94. What was learned from experiments in these two facilities, both of which study the combustion of liquid fuels, is summarized briefly here.
Liquid hydrocarbons supply the majority of the energy utilized in transportation. In North America last year we utilized about 2.5 tonnes of liquid fuels per capita, or about 2.5 times the world average. Combustion in general is the major methodology for converting the chemical energy in these fuels to useful thermal and/or mechanical forms. Combustion is also a major contributor to air pollution, including nitrogen oxides, carbon monoxide, unburned hydrocarbons, and particulates (we normally think of these as "soot" or "smoke"). In addition, carbon dioxide - a "greenhouse gas" - also is produced by the combustion of hydrocarbons.
It is relatively easy to produce a highly efficient conversion of the chemical energy in the fuels to thermal energy when there are no imposed constraints of the emissions which are produced. It is much harder to optimize the conversion of the thermal energy to useful mechanical energy, particularly with minimum emissions. Minimized emissions and the best "miles per gallon", if you will, require us to carefully control and tailor the combustion process. Whereas in earlier times, much of this could be accomplished by solely experimental R & D, today, very sophisticated computational design tools are necessary to guide this R & D. However, even small improvements in the fuel conversion to useful work with reduced emissions has very large economic impact on the economies of our nation and the world, as well as on both national, international, and global health issues. All of the fundamental combustion experiments aboard MSL-1 impact the fundamental sciences necessary to construct better computational design tools for internal combustion engines, including those in trucks, cars and aircraft, as well as in the industrial and electrical utility sectors. The droplet Combustion Experiment (DCE) and Filament Supported Droplet Combustion Experiment (FSDC-2) which we have aboard MSL-1 are unique in that they address these science issues for the large hydrocarbon molecular structures characteristic of those found in the liquid fuels utilized in the transportation sector.
The DCE and FSDC experiments both study isolated single droplet combustion phenomena, but operate in quite different ways. We have used the DCE to investigate the isolated droplet combustion behavior of a single liquid fuel, n-heptane, in a Helium-Oxygen gaseous environment at three different pressures. Both free-floating and tethered droplets can be studied in DCE. We were able to obtain all of the science we planned prior to the mission, and through some modified procedures put in place near the end of the mission, we were also able to study combustion in the shuttle cabin air admitted into the combustion chamber.
FSDC operates in the Mid-Deck Glove Box Facility, and can only use cabin air as the combustion environment. However, this experiment can utilize a number of different fuel types. In the present experiments, we studied methanol, two methanol water mixtures, ethanol, n-heptane, n-decane, and two mixtures of n-heptane/n-hexadecane. Experiments are much less sophisticated than those which can be conducted in DCE, but give us "first looks" at combustion behavior in microgravity. In addition to yielding some important science over a more limited range of parameters for each fuel, results are extremely helpful in developing test conditions for future work that can be conducted using DCE. The crew conducted our experiments so efficiently, that we able to not only study single droplet combustion on tethers, but we also conducted the first experiments in space on the combustion interactions of two droplets.
Both experiments use only very small amounts of liquid fuels (1/6 ounce for ALL of the DCE tests, 55; 1/60 ounce for each type of fuel studied in FSDC, or again, about 1/6 of an ounce of liquid for ALL of the FSDC tests).
DCE Experiments
Theories over the last 40 years have qualitatively predicted many of the phenomena to be observed in isolated droplet burning. Microgravity experiments allow us to quantitatively test these theories and develop quantitative models to interpret the results and predict behavior with reasonable accuracy at conditions where measurements have not been made. The sub-elements of these predictive models are transferable, with careful simplifications, to engineering design codes for practical energy conversion systems, such as internal combustion engines and gas turbines.
For example, theory using infinite-rate assumptions for chemical kinetics would tell us that in the DCE experiment, which burns free heptane droplets in helium-oxygen mixtures, we should likely see three regimes of droplet combustion. In addition to the well-known quasi-steady regime (which is frequently studied in ground-based experiments) two other regimes should exist which are transient in character. While infinite rate asymptotics qualitatively defines these transient regimes, there has been no experimental documentation of their existence. No models have existed to predict these issues quantitatively. As part of Princeton contribution to these projects, quantitative models involving detailed transport and chemical kinetics are under development and will be refined and tested with the data from MSL-1 experiments.
In the quasi-steady regime, all models show that the squares of both droplet and flame diameters decrease linearly with time and that diffusive extinction of the flame occurs when the droplet becomes sufficiently small, with a drop continuing to exist when the flame extinguishes. The DCE experiments aboard STS-94 not only further document this regime, but quantitatively show evidence of the two transient regimes noted above. For sufficiently large droplets, there is a transient regime, that of radiative extinction, in which energy loss by emission of infrared radiation from hot combustion products and soot causes flame extinction during the initial stage of flame expansion, leaving a large droplet that slowly vaporizes in the hot gases left behind after combustion has ceased. At sufficiently high oxygen content, there is another transient regime. Using infinite-rate kinetics, extinction of the flame is predicted to occur after the droplet has been fully vaporized completely. At extinction, the flame rapidly contracts, extinguishing diffusively at a non-zero flame radius. A transient regime near complete burnout of the droplet is observed in the DCE experiments which may be representative of this behavior. Further analyses of the data may show that the droplet does indeed disappear before the flame extinguishes, or that finite-rate chemistry causes the flame to extinguish before the droplet fully vaporizes. Without doubt, however, the DCE experiments quantify the two additional transient burning regimes for the first time.
To summarize all of our data for DCE 57 tests of 36 successful runs, we have obtained data for the following conditions:
- 1 Atm. Pressure (Helium-Oxygen)
- 25 successful experiments
- 20% - 35 % oxygen
- 0.5-4 mm
- 9 of the tests from MSL-1 STS-83 mission
- 0.5 Atm. Pressure (Helium-Oxygen)
- 14 Successful experiments
- 25% - 40 % Oxygen
- 1.5- 4.0 mm
- 1.5 mm drops were beyond the ignition limit in both 25% and 30% Oxygen
- 0.25 Atm. Pressure (Helium Oxygen)
- 2 Successful experiments
- 2.0 mm, 50% Oxygen
- 3.0 mm, 35% Oxygen
- Cabin Air (Nitrogen/21.8% Oxygen), 1 Atm. Pressure
- Two free drops (2 mm, 3 mm)
- Two tethered (75 micron silicon fiber) drops, (3 mm, 4 mm)
FSDC Experiments
FSDC-2 planned to conduct 52 total experiments, using nine different fuels or fuel mixtures. These were pure methanol, 85% methanol/15% water, 70% methanol/30% water, pure ethanol, 96% ethanol/4% water, n-heptane, n-decane, 95% n-heptane/5% n-hexadecane, and 80% n-heptane/20% n-hexadecane. Each experiment was to have involved the combustion of a single isolated droplet of between 2mm and 6 mm in diameter in cabin air. The FSDC-2 experiments were run in the Mid-Deck Glove Box (MGBX)Facility. The FSDC-2 experiments were performed over the early part of the mission, and all fuel supplies were fully expended as of July 12, 1997. Although the nominal mission was to have used eleven fuel cartridges, all 14 fuel cartridges were utilized to perform experiments. Each cartridge contains approximately 0.5 ml or about 1/60 of an ounce of liquid. One-hundred-twenty-seven (127) experiments were conducted, of which 123 resulted in successful tests. In addition to investigating the full range on initial droplet sizes with each fuel, seventeen experiments were conducted using two droplets, spaced about 1 cm apart, to study droplet-droplet interactions during combustion. These experiments yielded science that was originally planned for the flight of FSDC-1 on the STS-73 USML-2 mission in 1995.
The two drops are forced apart by ignition process, and then as the drops burn, they are forced together by the reduced vaporization on the adjacent sides of the drop. We named this effect, the "Thomas Twin Effect", after Don Thomas who was operating the experiment.
Acknowledgments
We want to especially thank the members of the STS-94 crew who conducted experiments on FSDC and DCE for us, notably, Roger Crouch, Greg Linteris, Don Thomas, and Janice Voss, and the cadre at MSFC which provided terrific support for our experiments during the mission. Forman Williams and I, for DCE, and in addition, Ben Shaw, John Haggard, Vedha Nayagam, for FSDC, are especially grateful as well to the other Science team members (Ron Collantonio, Anthony Marchese, Bei-Li Zhang) and the Engineering team members from NASA-Lewis who have assisted in controlling the operation of DCE and FSDC-2 during this mission.
Finally, I personally wish to make special note as a faculty and alumni from Princeton, that as we are concluding the celebration of our 250th birthday, this flight has been especially memorable for us. There have been more than 10 Princeton Faculty, former faculty, graduate and undergraduate alumni involved in this mission, including Dr. Greg Linteris, aboard STS-94.
Fred Dryer
Princeton University
July 18, 1997![]()
Movies
- MPEG Movie 1 of DCE Experiment
- MPEG Movie 2 of DCE Experiment
- MPEG Movie 3 of DCE Experiment
- MPEG Movie 4 of DCE ExperimentDroplet on a 75 micron fiber, in Cabin air.
- MPEG FSDC-2 Experiment 5.0 mm n-heptane Drop in Cabin Air.
Droplet Combustion Experiment ModuleInternal Apparatus Photographs appear elsewhere.
![]()
DCE Team MembersIn Photo(from left to right): Renato Colantonio (Project Deputy Scientist), Julie Grantier (Systems), John Haggard (Systems/Operations and Project Engineer), Vedha Nayagam (Project Scientist), Fred Dryer (Co-Investigator), Forman Williams (Principal Investigator), Tony Johnson (Operations), Steve Sholtis (Operations).
Not Shown: Neil Adams (Flight Software), Brian Borowski (Systems/Internal Apparatus), Gary Cartee (Operations), Dan Catalano (Internal Apparatus), Art Disantis (Internal Apparatus/Film Magazines), Mike Garrett (Avionics), Mike Hahn (Optics), Don Kodger (Operations/Data), John Kolacz (Avionics), Steve Lawn (Optics), Scott Lawyer (Avionics), Mark Lewis (Systems), Mike Lewis (Optics), Anthony Marchese (Science), Craig Myhre (Systems), Frank Novak (PGSC/Ground Software), Joe Ogonek (Flight/Ground Software), Mike Politi (Fuel Dispenser/Internal Apparatus), Mary Palumbo (Systems), Joe Ponyik (Software), Bill Schoren (Flight Safety), John Snead (Resources), Kevin Stultz (Optimus/Video Analysis), Bill Thompson (Ground Software), Rick Wiedenmannott (Test Chamber), Lowell Wolfe (Systems), Sylvia Yang (Avionics), and Bai Li Zhang (Science).
Other Links of Interest .
- At NASA:
Marshall Space Flight Center
MSL-1 Science Archive
Mission Science Highlights
STS-94 Mission Images
- At University of California at San Diego
![]()
![]()