At many colleges, students who are interested in science will enroll in multiple introductory courses, learning about genetics and chemistry and physics and computer programming as separate and largely unrelated disciplines. At Princeton, students have another option: the Integrated Science Curriculum (ISC). Four courses co-taught by multiple professors make up the integrated science curriculum — two classes in the fall, two in the spring.
“The labs have really been comprehensive,” said first-year student Bianca Swidler. “I feel like there’s been a great coverage of chemistry, physics, biology — it’s really hard to pick a favorite, like picking a favorite kid.” The photos below show ISC classroom and laboratory sessions. Each lab is spread across two weeks, to allow students time to learn new techniques and become comfortable applying them to research questions. Everyone pictured is a first-year student unless otherwise noted.
Lab 1: Reynolds numbers
Students drop spheres through clear liquids — a thick glycerin the first week, then water the second week — to measure the spheres’ falling speeds and calculate the Reynolds number of each fluid, a measure of its inertia and viscosity. In addition to introducing students to lab materials and techniques, the lab gives students an intuitive sense of how bacteria move through their thick, wet world. When students drop low-density aluminum balls through glycerin, the slow movement can be measured with just a ruler and a stopwatch. The students also use a motion-capture camera connected to lab computers to track the spheres’ descent from one frame to the next. The first week, they compare the computer-assisted measurements against those taken by hand. By the second week, when they drop steel balls through water, students depend on the camera and computer, as the dense beads fall quickly.
Lab 2: Electronics and solar power
During the first week of this two-part lab, students gain a fundamental grasp of circuitry. As their skills progress, the challenges get harder. By the second week, students make a nanocrystalline solar cell and build a circuit that can redirect the solar energy once it crosses a predetermined threshold. “It’s combining a little bit of chemistry and solar-cell engineering with circuitry and the physics behind that,” said Jennifer Gadd, a lecturer in chemistry and the Lewis-Sigler Institute for Integrative Genomics (LSI) who has been an ISC lab instructor for five years.
Lab 3: Fluorescent E. coli
In their first biology-focused lab, students examine the relationship between DNA (genes) and gene expression. They are given five “mystery samples” of E. coli bacteria, four of which carry the genetic instructions to produce mCherry, a fluorescent protein. As they work to identify their mystery samples, students must isolate DNA, run polymerase chain reactions (PCRs) to amplify the number of specific DNA segments, and use a fluorescent microscope.
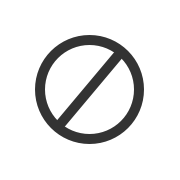
“A number of us at Princeton believe that some of the most exciting science of the future will take place at the boundaries between the traditional scientific disciplines,” said Joshua Shaevitz, a professor of physics and LSI, who is one of the curriculum’s several professors. “We hope to train a new generation of young scientists who naturally bridge these topics, feeling equally at home deriving equations, working with living cells at the bench and programming sophisticated computer analysis algorithms.”
He added: “Conventional introductory science classes can obscure the connections between fields by using different language and symbols for the same quantities or concepts. Integrated science is our attempt to teach a comprehensive introduction to the scientific endeavor that stresses the links between disciplines in a mathematically rigorous way.”
The curriculum was first imagined about 15 years ago, when several faculty members got together to create a cohesive introduction to the natural sciences. “Part of the idea was, ‘How can we convey the depths of the different subjects while encouraging the students to see their potential, in the broadest possible terms?’” said William Bialek, the John Archibald Wheeler/Battelle Professor in Physics and LSI, one of the creators of the integrated curriculum. “If you wait too long to introduce students to that way of thinking, you make their job more difficult, and you make our job more difficult.”
By the end of the integrated science curriculum, the students have received an unusually thorough preparation, said co-instructor Quan Wang, an associate research scholar at LSI and lecturer in physics and LSI who has also taught at Stanford University and the University of New Mexico.
“Last year, one of the students from this class ended up working in my lab,” Wang said. “It was really amazing to see a freshman who had developed so many skills just from this class that are directly integrate-able into modern-day research. It was something I’ve never seen before.”