This animation shows the snow and ice melt during a record heat wave in February 2020 on Eagle Island, near the northern tip of the Antarctic Peninsula. NASA Earth Observatory images by Joshua Stevens, using Landsat data from the U.S. Geological Survey and GEOS-5 data from the Global Modeling and Assimilation Office at NASA GSFC.
Princeton’s vital research across the spectrum of environmental issues is today and will continue to be pivotal to solving some of humanity’s toughest problems. Our impact is built on a long, deep, broad legacy of personal commitment, intellectual leadership, perseverance and innovation. This article is part of a series to present the sweep of Princeton’s environmental excellence over the past half-century.
Enter the front doors of Guyot Hall, the 111-year-old building that houses the Department of Geosciences at Princeton. Pass the glass specimen cases and the lobby’s iconic model of planet Earth and head to room M56. There, beyond the rows of heavy-duty snow boots and bulky parkas, stands a walk-in freezer storing some of the rarest artifacts of modern climate science: ancient ice cores harvested from Antarctica. At more than 2 million years old, these are the oldest ice cores ever collected.
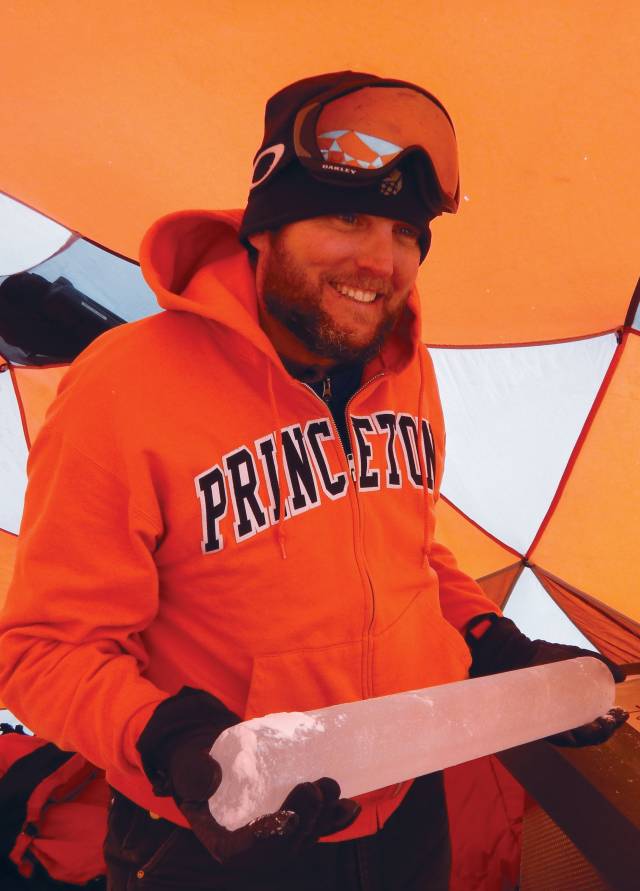
John Higgins
“Over the last 60-plus years, ice cores have produced the best evidence we have that carbon dioxide is linked to the Earth’s climate” said John Higgins, project leader for the group that recovered the ice in 2019 and an associate professor of geosciences.
“When we had an ice age, atmospheric carbon dioxide was significantly lower than it is today, and every time we didn’t have an ice age, atmospheric carbon dioxide was high — all that is known from ice cores,” he said. “My team is contributing to that puzzle by extending that record further back in time.”
The ice core research is emblematic of Princeton’s approach to climate science across the board over the last half-century, said Bess Ward, the William J. Sinclair Professor of Geosciences and the Princeton Environmental Institute (PEI) and chair of the geosciences department. “There's a saying that geoscientists believe firmly, which is, ‘The key to the present is the past, and the key to the future is the present,’” she said. “If we can understand the past, we can understand the future.”
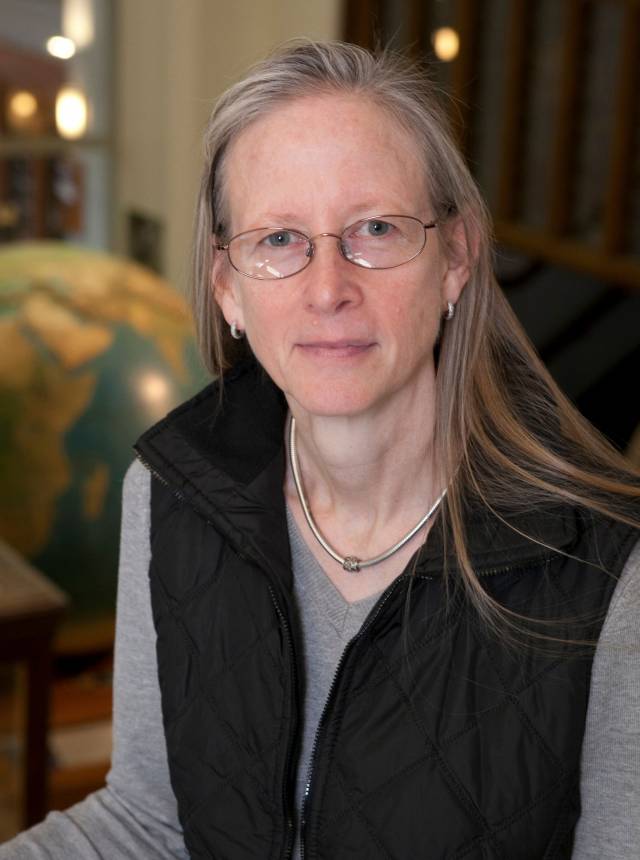
Bess Ward
And for more than 50 years, Princeton researchers have been doing just that. They’ve pushed back the boundaries of climate knowledge across a wide range of lynchpin issues. Princeton climate modelers, for example, developed the world's first coupled ocean-atmosphere model, using physical laws and present Earth conditions to develop mathematical algorithms that can predict how Earth’s climate will respond to different conditions in the future — and to understand what drove climate changes in the past. Funneling data into the models are Princeton oceanographers and field geologists who have fanned out across the globe to understand what oceans and ecosystems are doing today. And paleoclimatologists have been using fossils, pollen records, ice cores and other tools to study how the global climate has already changed in the planet’s long history.
Ground-truthing the theory
The combination of theory and observation has been critical. The importance of “boots on the ground, boats in the water” can’t be overstated, said Gabriel Vecchi, a leading climate modeler as well as a professor of geosciences and PEI. Modern climate modelers such as himself benefit from the direct observations gathered by the geologists and oceanographers working “just down the hall,” he said.
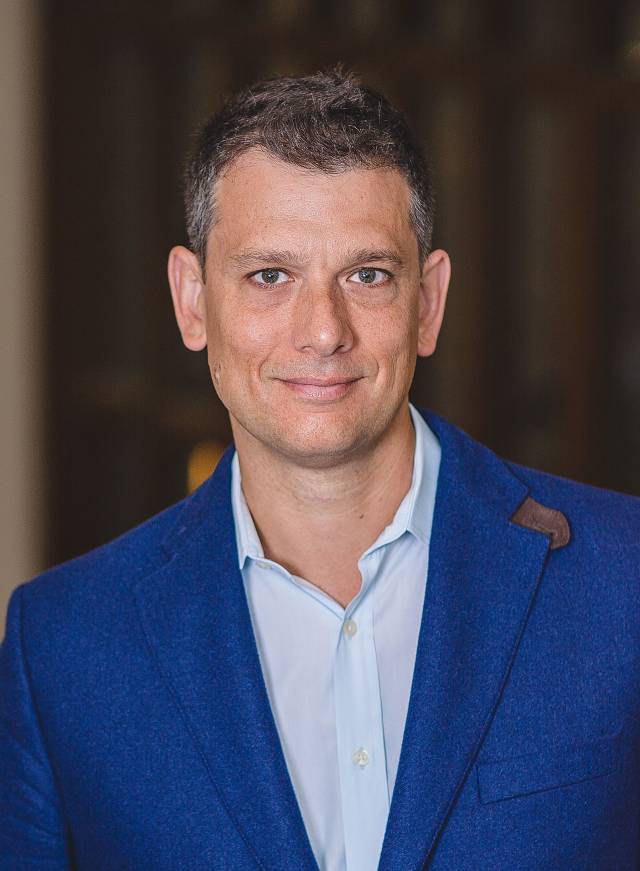
Gabriel Vecchi
“We can have great theories that make very nice predictions, but we need to test those predictions, and you only test those predictions with observations,” Vecchi said. “At the same time, the fundamental processes that we put into our models have to be developed from some sort of empirical base. So, both on the front end and the back end of building models, you need to have very strong involvement of observations.”
One Princeton scholar deeply involved in both theory and observation is Samuel G.H. “George” Philander, best known for his work on tropical oceans. His discovery of the recurring La Niña weather pattern and his seminal work on the related El Niño phenomenon dramatically improved scientists’ understanding of those enormous climate fluctuations. That knowledge, in turn, helps governmental and economic planners prepare for their effects.
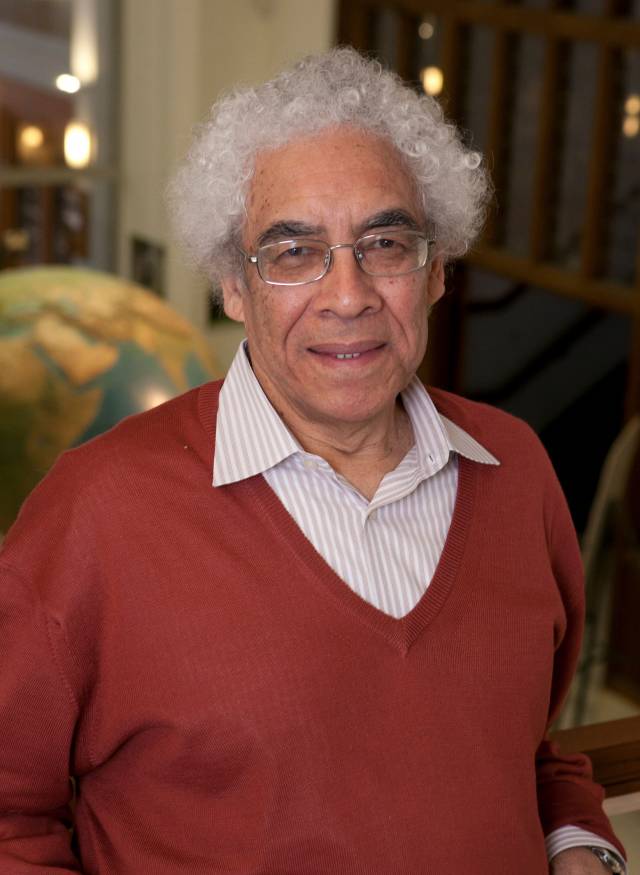
Samuel G.H. “George” Philander
Philander, now the Knox Taylor Professor of Geosciences, Emeritus, also helped organize a decade-long (1985-1994) international observational program known as TOGA, or Tropical Ocean Global Atmosphere. TOGA was designed to test the emerging theory of what is called “the coupled ocean-atmosphere system” in the tropics and paved the way for future ocean observation systems. It also led to improved simulations in global climate models.
“George was fundamental in developing our basic understanding of the El Niño phenomenon, which is the largest year-to-year fluctuation in the climate system,” said Vecchi. For hundreds of years, El Niño had been known as an ocean phenomenon that warmed the seas off the coast of Peru and shifted rainfall and other climate patterns. Philander helped shift scientists’ understanding of it from a purely oceanic event to one that was dependent on the coupled ocean-atmosphere system — the planet’s interconnected and interdependent water and air.
“That was a fundamental shift in the way that we looked at these fluids,” said Vecchi. “We realized that the ocean and atmosphere had to be understood together.”
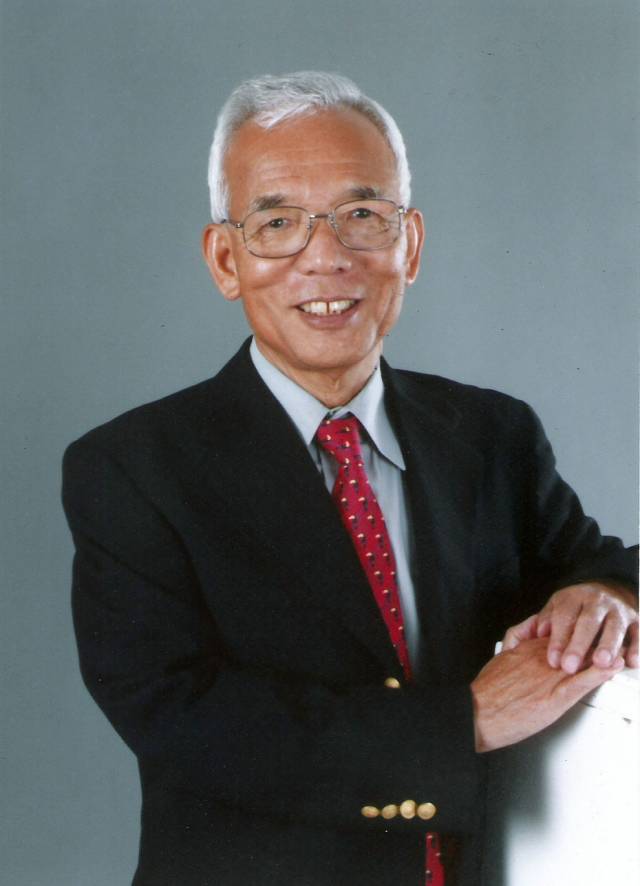
Syukuro Manabe
Also critical to that understanding was Syukuro “Suki” Manabe, one of the founders of modern climate modeling, who created the first coupled ocean-atmosphere computer model in 1969 with his colleague Kirk Bryan, an oceanographer. Both Manabe and Bryan were lecturers with the rank of professor at Princeton while also holding positions at the Geophysical and Fluid Dynamics Laboratory (GFDL) on Princeton’s Forrestal Campus. “An improved version of that coupled model has become indispensable not only for predicting the climate change of the industrial present but also for exploring the climate of the geological past,” Manabe said.
By changing the conditions (i.e., distribution of continent, concentration of carbon dioxide) modelers can recreate past eras as they predict the future, Manabe explained. Testing climate models against paleoclimates — ancient climates — is one of the key ways that climate modelers test their algorithms.
Frozen in time
The ice cores in Guyot Hall include many samples in addition to the multi-million-year-old record setter. The long, narrow cylinders of glacial ice, painstakingly gathered over the past half-century, are speckled with tiny bubbles of air that are trapped in the ice like dragonflies in amber. How do these trapped air bubbles form? As snow falls, it creates air pockets, and as that snow compacts into ice, those pockets become time capsules holding ancient air, whatever gases were present in the atmosphere, and even microscopic particles of pollen or volcanic ash — all clues to the past climate.
“For somebody who’s trying to reconstruct what the environment was like in the past, you can’t ask for anything better than a sample of ancient air, or a sample of ancient water,” said Higgins, who keeps breaking his own records for the oldest ice samples ever found. “In addition to the trapped air, you also have the ice itself, which provides its own record of climate at the time.”
Because they represent actual pieces of the past, instead of fossilized proxies, ice cores are considered the “gold standard” for paleoclimate studies, Higgins said. Climate scientists have built a record of the planet’s temperatures and carbon dioxide levels by measuring the gases and isotopes in these ancient samples. The continuous record goes back about 800,000 years before the present, and the older samples that Higgins has found provide snapshots of even earlier eras.
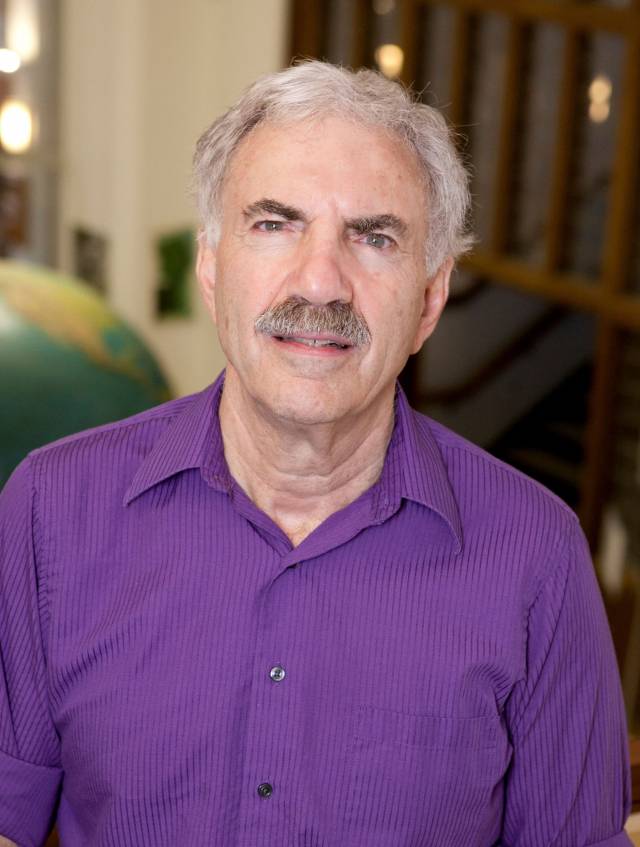
Michael Bender
Princeton has long been a leader in ice core research; Higgins came to the University to work with one of the founders of the field, Michael Bender, now an emeritus professor of geosciences. Bender is responsible for many of the innovations necessary to work with ancient ice, including a revolutionary approach to calculating the ice’s age by using the argon isotopes in the air bubbles.
“In truth, Michael has played a central role in the development of nearly all of the current activities on ice core gases and almost singlehandedly trained the field’s most prominent researchers,” said his colleagues when Bender transferred to emeritus status in 2014. “We can say unequivocally that he has been at the forefront of this pioneering field,” Higgins agreed.
In past decades, Bender was one of the scientists responsible for pushing the record back to 400,000 years, which included several ice age cycles. As Higgins and his team continued Bender’s work, they first extracted a 1-million-year old core in 2015 and then the 2+ million-year-old core in 2019.
The team is returning next season to look for even older samples. The goal is to find frozen bubbles of ancient atmosphere from a time period more than 2.7 million years ago that was 1-2 degrees warmer than today — a period often cited by climate scientists as a likely analogue for Earth’s climate in the 21st century. “Ice of this vintage would provide researchers with the first direct evidence for atmospheric greenhouse gases at that time and an unprecedented glimpse of many important aspects of Earth’s climate system,” Higgins explained.
“Reconstructing Earth’s climate in the past, studying climate change in the future — these are two complementary ways of getting at the same question,” Higgins said.
The ocean’s role in climate
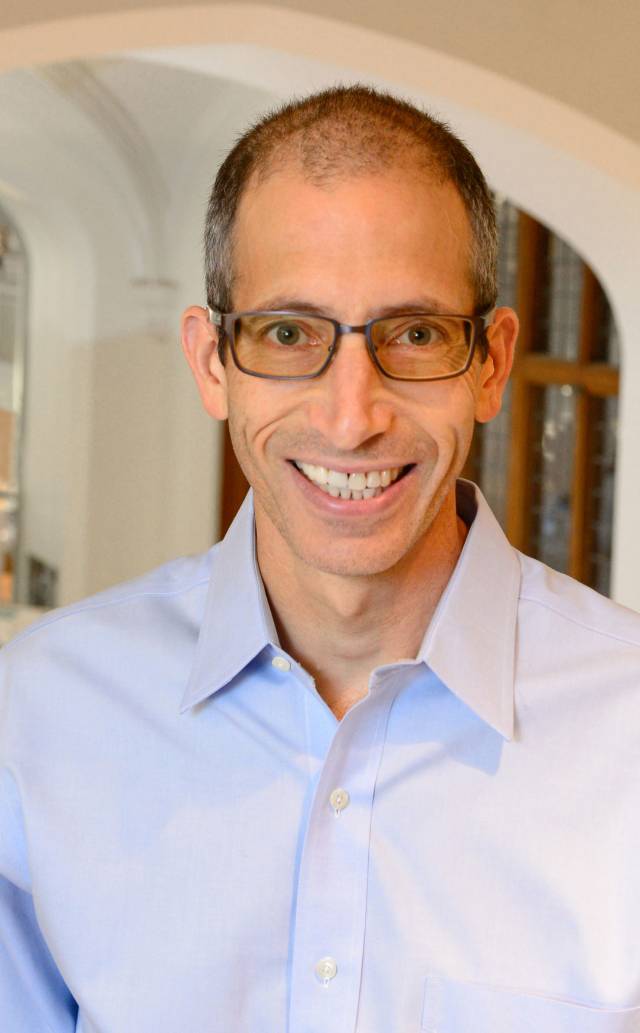
Daniel Sigman
Ice core bubbles can show carbon dioxide, methane and other greenhouse gases increasing during warm periods and dropping during ice ages, but they can't say why it happened. Figuring that out involves examining the carbon cycle from a variety of angles.
In Higgins’ view, “Understanding the carbon cycle involves all of our different gifts.” He cited the work of oceanographers Bess Ward and Daniel Sigman. Ward takes crews of students and other researchers to key locations around the world to investigate the global ocean’s nitrogen and carbon cycling. Sigman’s research group also makes new kinds of measurements in fossils from ocean sediment cores. The results reveal how past changes in ocean conditions have altered the storage of carbon dioxide in the ocean, changing atmospheric carbon dioxide levels and thus global climate.
“Danny Sigman's research has been at the forefront of understanding ancient oceans,” said Ward. “And by that, I mean, the circulation and the biological and chemical conditions of the oceans in the past. …Understanding the oceans of the past is the only way we can think to understand the oceans of the future. So if we understand how ocean circulation, for example, responded to changes in global temperature or distribution of temperature or distribution of ice, then we can have a good shot at forecasting what will happen when the ice caps melt.”
To study the world’s oceans, past and present, Ward and Sigman rely on sophisticated shipboard instrumentation and retrieval tools that drop deep below the ocean surface, far below levels that can be reached by scuba divers.
“The deep ocean is the ocean,” stressed Sigman, the Dusenbury Professor of Geological and Geophysical Sciences. Globally, the oceans have an average depth of about 3,657 meters (12,000 feet), while the “surface ocean” is generally defined as the top 100 meters (330 feet).
“The surface ocean is the thin skin,” he said. “It is the interface with the atmosphere and the habitat for most ocean life. It’s critical — but the biggest part of the ocean’s volume is the deep ocean. So the question becomes, how rapidly can heat from global warming and carbon dioxide from fossil fuel burning make their way into the deep ocean?”
Surveying the seas
Ocean cruises led by Ward and colleagues are necessarily limited in space and time, but Princeton researchers have also been leaders in the evolving field of remote data gathering.

Jorge Sarmiento
The flotilla of observational floats in the TOGA program that George Philander helped organize, paved the way for the Southern Ocean Carbon and Climate Observations and Modeling project (SOCCOM), a multi-institutional program housed at Princeton and funded by the National Science Foundation. It uses more than 150 floating data collectors to determine how the Southern Ocean — the ocean encircling Antarctica — influences the world’s climate. The project is directed by Jorge Sarmiento, a biogeochemist and the George J. Magee Professor of Geoscience and Geological Engineering, Emeritus.
In the 1980s, Sarmiento and GFDL scientist J.R. "Robbie" Toggweiler were one of three groups to simultaneously discover the importance of the Southern Ocean in controlling the atmosphere’s carbon dioxide levels. Since that work, a lack of data has hampered understanding of this critical but remote region. The SOCCOM project is a "game-changer," said Sigman, acquiring data even through the harshest of winter conditions. Sarmiento’s discoveries about the Southern Ocean have inspired Higgins and Sigman, who have looked to the Southern Ocean in the effort to explain past changes in atmospheric carbon dioxide and climate.
Princeton’s deep strength in ocean studies is no accident, said Ward. “Climate, as a subset of environmental research, is a motivating factor for all of the research that we do,” she said. “The reason we're not already at 4 degrees Celsius of global warming — or more — is because a substantial portion of the carbon dioxide that humans have emitted is now in the ocean. And so the biogeochemistry of the ocean becomes critical to understanding the biogeochemistry of the globe.”
For decades, Sarmiento was one of the only biogeochemists in the world — “I was kind of an orphan,” he joked — until more and more scientists came to see how interconnected the natural sciences are.
“If you want to study the natural world, you’re operating at the intersection between physics, chemistry, biology and geoscience,” said Sigman. “That’s what’s behind the snarl of a name: biogeochemistry. What attracted me to this work in the first place was that I didn’t want to leave any of these disciplines behind — and I haven’t had to.”